Research
1. Porous Adsorbents and Catalysts
Microporous zeolites with large internal surfaces of up to 2000 m2/g and pore or cage diameters in the range of 0.5 to 1.2 nm, e.g. for zeolites SAPO-34, SSZ-13, ZSM-5, MCM-22, EU-1,
Beta, and Y, are reaction vessels in a molecular scale and play an important role as shape-selective catalysts in research and chemical industry (see "Publications", Topic 1 of "NMR Methods for Heterogeneous Catalysis" and [1]). Substitution of framework silicon
by aluminum atoms makes these materials to solid acids, which are suitable to replace polluting liquid acids as catalysts in numerous industrial processes. Due to their well-defined pore systems, these materials are also utilized as molecular sieves in separation processes. During the past decades, routes for the synthesis of
mesoporous materials with pore diameters of more than 10 nm were developed. These mesoporous materials, such as MCM-41 ([2],
[3], [4],
[5]) and SBA-15 ([6], [7],
[8], [9]), have frameworks consisting of
amorphous walls and ordered mesopores in hexagonal arrangement. Since these materials have no intrinsic catalytic properties, modification of their internal surface is required for developing
novel mesoporous solids, which are interesting for applications as adsorbents and catalysts. Dry-gel synthesis routes, the use of zeolite
seeds in the synthesis of ordered mesoporous materials, and the introduction of metal species lead to hybrid catalysts with Broensted acidic ([4],
[10], [11], [12]), Lewis acidic
[5], and redox properties [13].
In further approaches, ordered mesoporous materials were utilized as supports of catalytically active
compounds, such as aluminum species ([3], [14],
[9]) and noble metals
([8], [15], [16]).
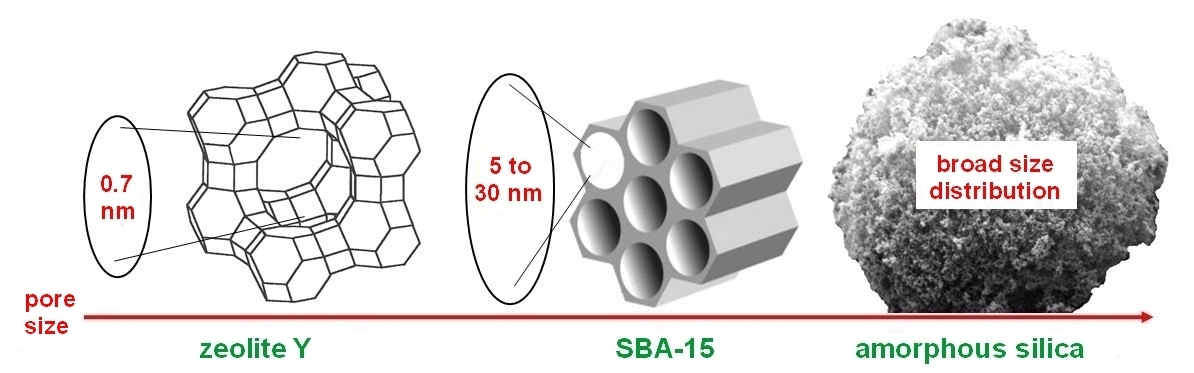 |
2. Local Structure and Properties of Surface Sites on Porous Adsorbents and Catalysts
Solid-state NMR spectroscopy (NMR: Nuclear Magnetic Resonance) is a very important analytical tool of materials science with high sensitivity for the short-range
order of solids. Most of the atoms contributing to the framework of adsorbents and solid catalysts applied in chemical
industry consist of isotopes with nuclear spin I. These isotopes open the way for applying
solid-state NMR spectroscopy to study framework atoms and surface sites, such as oxygen coordinations, atom-atom distances, bond angles,
atom densities, and atom dynamics. Two-dimensional techniques of NMR spectroscopy allow the separation of solid-state interactions and the study of their
influence on resonating nuclei. An important solid-state interaction is the quadrupolar interaction of nuclei with spin I > 1/2
with the electric field gradient caused by the charge distribution in their local structure. By analysing the parameters of the quadrupolar interaction, the oxygen coordination and the location of
sodium and aluminum species in zeolites are clarified ([17],
[18], [19]). By analysis of 1H NMR broad-line shapes or intensity distributions of 1H MAS NMR sideband patterns, and by TRAPDOR and REAPDOR experiments, the dipolar coupling between neighbouring atoms is evaluated and atom-atom distances are determined
([20], [21], [22],
[23]). In contrast to diffraction techniques, which are suitable for determining the long-range order of crystalline materials, solid-state NMR spectroscopy can also be applied for the investigation of amorphous materials ([24],
[25]). A very important topic is the characterization of the chemical properties of catalytically active sites inside the pores and at the outer surface of solids.
For this purpose, various methods for the solid-state NMR investigation of surface sites on adsorbents and catalysts without and with adsorption of probe molecules were developed and
improved (see "Publications", Topics 2 to 4 of "NMR Methods for Heterogeneous Catalysis" and [26],
[27], [28]). The spectroscopic results of
these investigations on Broensted and Lewis acidic surface sites as well as supported noble metals are utilized for improving our understanding of the chemical properties of the materials under study in their specific applications.
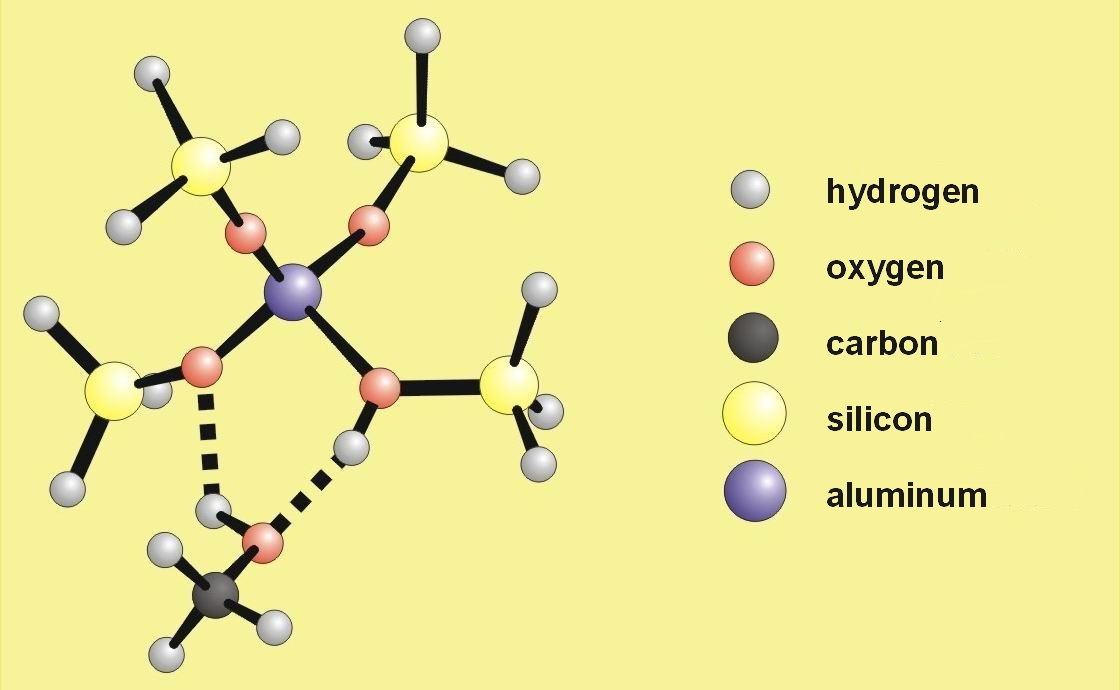 |
3. In Situ Solid-State NMR Spectroscopy of Working Catalysts
In situ solid-state NMR techniques are suitable for the
investigation of working solid catalysts under batch, semi-batch, stopped-flow, pulsed-flow, and continuous flow conditions (see "Publications", Topic 5 of "NMR Methods for Heterogeneous Catalysis" and [29]). By application of these in situ techniques, catalytically active sites,
adsorbate complexes, intermediates, and coke deposits on the surface of working solid catalysts are studied and reaction mechanisms and
deactivation processes occurring under industrially relevant conditions are elucidated [30].
Examples are investigations of the methyl tertiary-butyl ether synthesis ([31], [32])
and the conversion of methanol to dimethyl ether and olefins ([33], [34]) on acidic zeolite catalysts, the alkylation of toluene
([35], [36]), aniline
([37], [38]), and phenol [39]
on basic and acidic zeolites, and the hydrogenation of olefins [40] on noble metal containing solid catalysts.
In situ 13C MAS NMR spectroscopy of the conversion of methanol on zeolite H-ZSM-5 and on the crystalline
silicoaluminophosphates SAPO-18 and SAPO-34 under continuous-flow conditions support the formation of a catalytically active pool of
hydrocarbons in the catalyst pores and cages, which consists of a mixture of branched aliphatic and aromatic compounds [41].
After purging the spent MTO catalysts with pure carrier gas, polymethylaromatics were preferentially found in the pores of these zeolites. These
approaches give direct experimental evidence for the hydrocarbon-pool mechanism discussed in literature [42]. According to this
mechanism, carbonaceous species exist inside the zeolite pores and cages, which add reactants and split off reaction products, such
as light olefins [43].
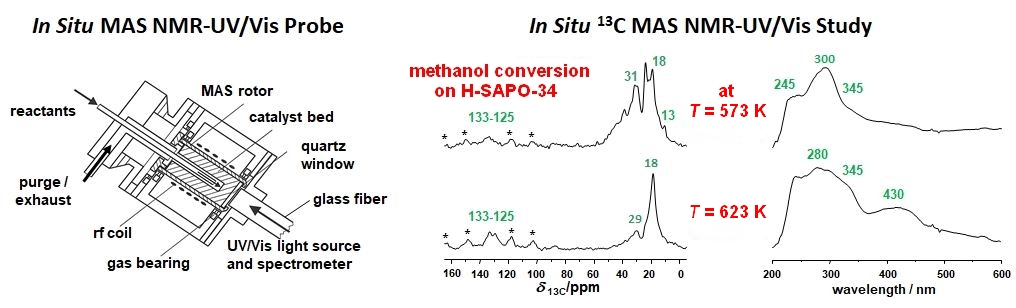 |
References
[1] J. Weitkamp, M. Hunger, Acid and Base Catalysis on Zeolites, in: J. Cejka, H. van Bekkum, A. Corma, F. Schueth (eds.),
Introduction to Zeolite Molecular Sieves, 3rd Edition, Stud. Surf. Sci. Catal., Volume 168, Elsevier, Amsterdam,
2007, ISBN: 9780080550411, p. 787-835.
[2] M. Hunger, U. Schenk, M. Breuninger, R. Glaeser, J. Weitkamp, Characterization of the acid sites in MCM-41-type materials by
spectroscopic and catalytic techniques, Microporous Mesoporous Mater. 27 (1999) 261-271, DOI: 10.1016/S1387-1811(98)00260-1.
[3] M. Xu, A. Arnold, A. Buchholz, W. Wang, M. Hunger, Low-temperature modification of mesoporous MCM-41 material with sublimated
aluminum chloride in vacuum, J. Phys. Chem. B 106 (2002) 12140-12143, DOI: 10.1021/jp021308a.
[4] M. Xu, W. Wang, J. Weitkamp, M. Hunger, Dry-gel synthesis of mesoporous MCM-41 materials with modified pore structure,
Z. Phys. Chem. 219 (2005) 877-890, DOI: 10.1524/zpch.219.7.877.67089.
[5] K.D. Kim, Z. Wang, M. Hunger, J. Huang, The cooperative effect of Lewis acid and Broensted acid on Sn-MCM-41 catalysts for the
conversion of 1,3-dihydroxyacetone to ethyl lactate, Green Chemistry 21 (2019) 3383-3393, DOI: 10.1039/C9GC00820a.
[6] V.R. Reddy Marthala, S. Rabl, J. Huang, B. Thomas, M. Hunger, In situ solid-state NMR investigations of the vapor-phase Beckmann
rearrangement of 15N-cyclohexanone oxime on MFI-type zeolites and mesoporous SBA-15 materials in the absence and
presence of the additive 13C-methanol, J. Catal. 257 (2008) 134-141, DOI: 10.1016/j.jcat.2008.04.014.
[7] J. Frey, Y.S. Ooi, B. Thomas, V.R. Reddy Marthala, A. Bressel, T. Schoelkopf, T. Schleid, M. Hunger, Vanadium phosphates on
mesoporous supports: Model catalysts for solid-state NMR studies of the selective oxidation of n-butane, Solid State
Nucl. Magn. Reson. 35 (2009) 130-137, DOI: 10.1016/j.ssnmr.2009.02.005.
[8] M. Dyballa, C. Rieg, D. Dittmann, Z. Li, M. Buchmeiser, B. Plietker, M. Hunger, Potential of triphenylphosphine as solid-state NMR
probe for studying the noble metal distribution on porous supports, Microporous & Mesoporous Mater. 293 (2020) 109778,
DOI: 10.1016/j.micromeso.2019.109778.
[9] Z. Li, C. Rieg, A.-K. Beurer, M. Benz, J. Bender, C. Schneck, Y. Traa, M. Dyballa, M Hunger, Effect of aluminum and sodium on the
adsorption of water and methanol in microporous MFI-type zeolites and mesoporous SBA-15 materials, Adsorption (2020),
DOI: 10.1007/s10450-020-00275-8.
[10] X. Vu, U. Bentrup, M. Hunger, U. Armbruster, A. Martin, Direct synthesis of nanosized-ZSM-5/SBA-15 analog composites
from preformed ZSM-5 precursors for improved catalytic performance as cracking catalyst, J. Mater. Sci. 49 (2014)
5676-5689, DOI: 10.1007/s10853-014-8287-z.
[11] L.T. Hoai Nam, T. Quang Vinh, N. Duc Hoa, M. Hunger, Synthesis and characterization of ZSM-5/SBA-15 composite material,
Int. J. Nanotechnology 12 (2015) 466-474, DOI: 10.1504/IJNT.2015.067904.
[12] X. Hoan Vu, M. Hunger, U. Armbruster, A. Martin, Influence of the initial Si/Al ratio on the structural, acidic and
catalytic properties of nanosized-ZSM-5/SBA-15 analog composites prepared from ZSM-5 precursors, J. Porous Mater. 25 (2018)
1035-1046, DOI: 10.1007/s10934-017-0514-y.
[13] J. Frey, C. Lieder, Th. Schoelkopf, Th. Schleid, U. Nieken, E. Klemm, M. Hunger, Quantitative solid-state NMR investigation of
V5+ species in VPO catalysts upon sequential selective oxidation of n-butane, J. Catal. 272 (2010) 131-139,
DOI: 10.1016/j.jcat.2010.03.004.
[14] S. Lang, M. Benz, U. Obenaus, R. Himmelmann, M. Scheibe, E. Klemm, J. Weitkamp, M. Hunger, Mechanisms of the AlCl3
modification of siliceous microporous and mesoporous catalysts investigated by multi-nuclear solid-state NMR, Top. Catal.
60 (2017) 1537-1553, DOI: 10.1007/s11244-017-0837-6.
[15] U. Obenaus, S. Lang, R. Himmelmann, M. Hunger, In situ MAS NMR investigation of parahydrogen induced hyperpolarization inside
meso- and micropores of Ir-, Pt-, Rh-, and Pd-containing solid catalysts, J. Phys. Chem. C 121 (2017) 9953-9962,
DOI: 10.1021/acs.jpcc.7b01899.
[16] Z. Wang, K.-D. Kim, C. Zhou, M. Chen, N. Maeda, Z. Liu, J. Shi, A. Baiker, M. Hunger, J. Huang, Influence of support
acidity on the performance of Pt nanoparticles in the chemoselective hydrogenation of acetophenone over Pt/[Al]MCM-41,
Catal. Sci. Technol. 5 (2015) 2788-2797, DOI: 10.1039/c5cy00214a.
[17] M. Hunger, G. Engelhardt, H. Koller, J. Weitkamp, Characterization of sodium cations in dehydrated faujasites and zeolite EMT by
23Na DOR, 2D nutation, and MAS NMR, Solid State Nucl. Magn. Reson. 2 (1993) 111-120, DOI: 10.1016/0926-2040(93)90029-M.
[18] M. Hunger, P. Sarv, A. Samoson, Two-dimensional triple-quantum 23Na MAS NMR spectroscopy of sodium cations in
dehydrated zeolites, Solid State Nucl. Magn. Reson. 9 (1997) 115-120, DOI: 10.1016/S0926-2040(97)00051-9.
[19] J. Jiao, J. Kanellopoulos, W. Wang, S. S. Ray, H. Foerster, D. Freude, M. Hunger, Characterization of framework and extra-framework
aluminum species in non-hydrated zeolites Y by 27Al spin-echo, high-speed MAS, and MQMAS NMR spectroscopy at B0 = 9.4 to 17.6 T,
Phys. Chem. Chem. Phys. 7 (2005) 3221-3226, DOI: 10.1039/b508358c.
[20] M. Hunger, D. Freude, D. Fenzke, H. Pfeifer, 1H solid-state NMR studies of the geometry of Broensted acid sites in zeolites H-ZSM-5,
Chem. Phys. Lett. 191 (1992) 391-395, DOI: 10.1016/0009-2614(92)85397-S.
[21] M. Hunger, M. Anderson, A. Ojo, H. Pfeifer, Study of the geometry and location of the bridging OH groups in aluminosilicate and
silicoaluminophosphate type zeolites using 1H MAS NMR sideband analysis and CP/MAS NMR, Microporous Mater.
1 (1993) 17-32, DOI: 10.1016/0927-6513(93)80005-F.
[22] S. Greiser, M. Hunger, C. Jaeger, 29Si{27Al}TRAPDOR MAS NMR to distinguishes Qn(mAl) sites in alumosilicates. Test case:
Faujasite-type zeolites, Solid State Nucl. Magn. Reson. 79 (2016) 6-10, DOI: 10.1016/j.ssnmr.2016.10.004.
[23] H. Koller, T. Uesbeck, M. R. Hansen, M. Hunger, First and second neighbors of Broensted and Lewis acid sites in zeolites and the
distribution of 27Al quadrupolar coupling, analyzed by 1H{27Al} offset REAPDOR, J. Phys. Chem. C 121 (2017) 25930-25940,
DOI: 10.1021/acs.jpcc.7b09544.
[24] Z. Wang, Y. Jiang, X. Yi, C. Zhou, A. Rawal, J. Hook, Z. Liu, F. Deng, A. Zheng, M. Hunger, A. Baiker, J. Huang, High population
and dispersion of pentacoordinated AlV species on the surface of flame-made amorphous silica-alumina,
Science Bulletin 64 (2019) 516-523, DOI: 10.1016/j.scib.2019.04.002.
[25] Z. Wang, Y. Jiang, F. Jin, C. Stampfl, M. Hunger, A. Baiker, J. Huang, Strongly enhanced acidity and activity of amorphous
silica-alumina by formation of pentacoordinated AlV species, J. Catal. 372 (2019) 1-7, DOI: 10.1016/j.jcat.2019.02.007.
[26] M. Hunger, Multinuclear solid-state NMR studies of acidic and non-acidic hydroxyl protons in zeolites, Solid State Nucl. Magn. Reson.
6 (1996) 1-29, DOI: 10.1016/0926-2040(95)01201-X.
[27] M. Hunger, Broensted acid sites in zeolites characterized by multi-nuclear solid-state NMR spectroscopy, Catal. Rev.-Sci. Eng. 39 (1997)
345-393, DOI: 10.1080/01614949708007100.
[28] Y. Jiang, J. Huang, W. Dai, M. Hunger, Solid-state nuclear magnetic resonance investigations of the nature, property, and activity of
acid sites on solid catalysts, Solid State Nucl. Magn. Reson. 39 (2011) 116-141, DOI: 10.1016/j.ssnmr.2011.03.007.
[29] M. Hunger, In situ flow MAS NMR spectroscopy: State of the art and applications in heterogeneous catalysis, Prog. Nucl. Magn. Reson.
Spectrosc. 53 (2008) 105-127, DOI: 10.1016/j.pnmrs.2007.08.001.
[30] Y. Jiang, J. Huang, V.R. Reddy Marthala, Y.S. Ooi, J. Weitkamp, M. Hunger, In situ MAS NMR-UV/Vis investigation of H-SAPO-34
catalysts partially coked in the methanol-to-olefin conversion under continuous-flow conditions and of their regeneration, Microporous
Mesoporous Mater. 105 (2007) 132-139, DOI: 10.1016/j.micromeso.2007.05.028.
[31] M. Hunger, T. Horvath, J. Weitkamp, Methyl tertiary-butyl ether synthesis on zeolite HBeta investigated by in situ MAS NMR
spectroscopy under continuous-flow conditions, Microporous Mesoporous Mater. 22 (1998) 357-367,
DOI: 10.1016/S1387-1811(98)00078-X.
[32] T. Horvath, M. Seiler, M. Hunger, A comparative study of methyl-tert-butyl ether synthesis on zeolites HY, HBeta, HBeta/F and
HZSM-5 by in situ MAS NMR spectroscopy under flow conditions and on-line gas chromatography, Appl. Catal. A:
General 193 (2000) 227-236, DOI: 10.1016/S0926-860X(99)00432-9.
[33] W. Wang, M. Seiler, M. Hunger, Role of surface methoxy species in the conversion of methanol to dimethyl ether on acidic zeolites
investigated by in situ stopped-flow (SF) MAS NMR spectroscopy, J. Phys. Chem. B 105 (2001) 12553-12558,
DOI: 10.1021/jp0129784.
[34] W. Wang, A. Buchholz, M. Seiler, M. Hunger, Evidence for an initiation of the MTO process by reactive surface methoxy groups on acidic
zeolite catalysts, J. Am. Chem. Soc. 125 (2003) 15260-15267, DOI: 10.1021/ja0304244.
[35] M. Hunger, U. Schenk, J. Weitkamp, Mechanistic studies of the side-chain alkylation of toluene with methanol on basic zeolites Y by
multi-nuclear NMR spectroscopy, J. Mol. Catal.: A 134 (1998) 97-109, DOI: 10.1016/S1381-1169(98)00026-0.
[36] M. Hunger, U. Schenk, M. Seiler, J. Weitkamp, In situ MAS NMR spectroscopy of surface compounds formed by conversion of methanol
and a toluene/methanol feed on basic zeolite X under batch and flow conditions, J. Mol. Catal. A: Chemical 156 (2000) 153-161,
DOI: 10.1016/S1381-1169(99)00404-5.
[37] W. Wang, M. Seiler, I.I. Ivanova, J. Weitkamp, M. Hunger, In situ stopped-flow (SF) MAS NMR spectroscopy: A novel NMR technique
applied for the study of aniline methylation on a solid base catalyst, Chem. Commun. (2001) 1362-1363, DOI: 10.1039/b104115k.
[38] I.I. Ivanova, E.B. Pomakhina, A.I. Rebrov, M. Hunger, Yu.G. Kolyagin, J. Weitkamp, Surface species formed during aniline methylation
on zeolite H-Y investigated by in situ MAS NMR spectroscopy, J. Catal. 203 (2001) 375-381, DOI: 10.1006/jcat.2001.3300.
[39] W. Wang, P.L. De Cola, L. Glaeser, I.I. Ivanova, J. Weitkamp, M. Hunger, Methylation of phenol by methanol on acidic zeolite H-Y
investigated by in situ CF MAS NMR spectroscopy, Catal. Lett. 94 (2004) 119-123, DOI: 10.1023/B:CATL.0000019341.67169.ac.
[40] U. Obenaus, F. Neher, M. Scheibe, M. Dyballa, S. Lang, M. Hunger, Relationships between the hydrogenation and dehydrogenation
properties of Rh-, Ir-, Pd-, and Pt-containing zeolites Y studied by in situ MAS NMR spectroscopy and conventional heterogeneous
catalysis, J. Phys. Chem. C 120 (2016) 2284-2291, DOI: 10.1021/acs.jpcc.5b11367.
[41] M. Hunger, M. Seiler, A. Buchholz, In situ MAS NMR spectroscopic investigation of the conversion of methanol to olefins on
silicoaluminophosphates SAPO-34 and SAPO-18 under continuous flow conditions, Catal. Lett. 74 (2001) 61-68,
DOI: 10.1023/A:1016687014695.
[42] M. Dyballa, P. Becker, D. Trefz, E. Klemm, A. Fischer, H. Jacob, M. Hunger, Parameters influencing the selectivity to propene in the MTO
conversion on 10-ring zeolites: Directly synthesized silicoalumino-type zeolites ZSM-5, ZSM-11, and ZSM-22, Appl. Catal. A:
General 510 (2016) 233-243, DOI: 10.1016/j.apcata.2015.11.017.
[43] X. Wang, W. Dai, G. Wu, L. Li, N. Guan, M. Hunger, Verifying the dominant catalytic cycle of the methanol-to-hydrocarbon conversion
over SAPO-41, Catal. Sci. Technol. 4(3) (2014) 688-696, DOI: 10.1039/c3cy00740e.
Michael Hunger / Stuttgart, Germany
home